

History of Superfluorescence
Albert Einstein Professor in Science at PU (1975-1984)
•Dicke Superradiance (1954)
https://journals.aps.org/pr/pdf/10.1103/PhysRev.93.99
Since then……
Bose- Einstein Condensates (1973)
•Plasmonics (Pustovit et al., Proc. SPIE 7757, Plasmonics: Metallic Nanostructures and Their Optical Properties VIII, 77571U (10 September 2010))
•Semiconductor Quantum Structures (G. Noe ll et al., Nature Physics 8, 219 (2012))
•Quantum Dot Superlattice (G. Raino et al., Nature 563, 671–675 (2018))
•Until recently, needs extremely low T (6K to 100K or high magnetic fields >10T) to suppress dephasing.
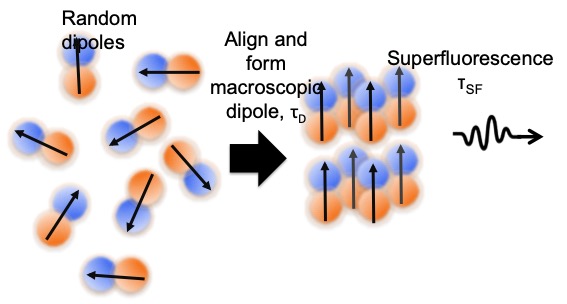
What is Superfluorescence?
•Short and intense burst of light.
•Pulse peak intensity scales as N2 and a lifetime that scales as tSF ~ tSP/N .
•Disrupted by phase relaxation.
No previous reports of Anti-Stokes shifted SF has been observed, at ambient temperature, and in a single nanocrystal until our breakthrough.

•4f orbitals of lanthanide (RE3+) ions shielded by the outer fully-occupied 5s and 5p.
•Each closely spaced RE3+ emitter interact with each other through the radiation field to establish coherence
•Anti-Stokes Superfluorescent Emission at RT
Overview of Quantum Optics Research
Superfluorescence (SF) is a collective quantum mechanical optical emission arising from the self-organization between nano-emitters, forming a super dipole coupled with its own radiation field (resulting in the characteristic Burnham Chiao ringing). In contrast to spontaneous emission from an ensemble of incoherent emitters or conventional fluorescence, SF produces a short intense burst of highly correlated photons, making it ideal for applications in photonics, potentially as a photon source for quantum communication and information processing.
Until our recent work, SF required cryogenic temperatures, so the properties of the SF photon correlations remain untested and underappreciated. We have observed both coherent short pulses and ringing pulses simultaneously at room temperature. Our SF system’s additional optical ringing effect provides not only frequency but also time information.

The ability to control the short pulse emission wavelength (via dopants), lifetime, and ringing periodicity opens up new applications inaccessible to conventional sources. Potential applications include encryption and optical timer/computing purposes, where the lack of EMI is particularly crucial for preventing hacking.
Overview of Nanophotonics Research
We are working on understanding the physics of nanophotonic materials and their applications in biosensing and solar cell devices. We optimize our proposed nanostructures with predictive finite element modeling, and perform correlated structural and optical single nanoparticle spectroscopy to explore the link between nanoparticle size/geometry/orientation and its corresponding optical property. As such, we use analytical tools (AFM, fluorescence microscopy, single particle time-resolved spectroscopy, SEM, TEM) and other experimental methods in order to elucidate the nanostructure effect on photophysical mechanisms.

Nanoplasmonic Upconverting Nanoparticles as Orientation Sensors for Single Particle Microscopy
Recent work from my lab has demonstrated a novel application of UCNPs as a unique 3D tracker. By coating disk-shaped UCNPs with a metal layer (NP-UCNP), they gain a large anisotropy in the fluorescence yield if illuminated with polarized light. This fluorescence anisotropy of the NP-UCNP probe renders them as excellent orientation probes in both linear and 3D tracking, which allow the continuous tracking of single-molecules in a variety of settings. Our experimental observations were supported by finite element calculations, showing that the change in fluorescence intensity with nanoparticle orientation is dependent on the geometry of the NP-UCNP. Additionally, we have observed at the single particle level, a change in fluorescence intensity with excitation polarization. Our calculations show that this effect is attributed to the anisotropic disk shape of the nanocrystal, which gives rise to non-uniform dipole orientations along crystal axes.
Enhancement of Upconverted Fluorescence by Interference Modulation
Work in my lab have shown, that as an alternative to plasmonic enhancement, interference modulation provides similar levels of absorption and fluorescence enhancement, without the precise control in spacer layer thickness normally required in optimal plasmonic enhancement. Our experimental and calculated results show the influence of the interference modulation on both the excitation (interion energy transfer) and radiative decay with nonradiative decay processes active between emissive levels in the upconversion system. We have compared measured single particle and calculated spectra, showing agreement of emission intensity enhancements, at the optimal spacer layer/gold mirror configuration. This shows that the emission enhancement is a combination of the enhanced near infrared absorption and also the change in the transition probabilities in both green and red emissions with coupling to the spacer/gold mirror configuration. This particular configuration shows high tolerance in the spacer thickness variation, and the planar design, leads to ease in implementation in device applications.
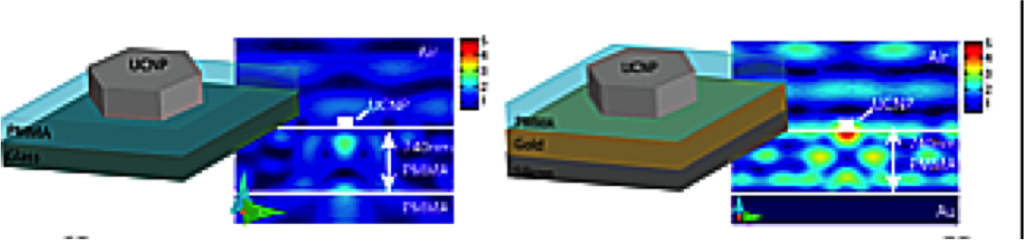
Plasmonic Enhancement of Upconversion Emission With A Gold Shell
Upconversion of infrared light to visible light has important implications for single molecule bioimaging and tracking. Hence, investigation of upconversion at the single particle level is necessary for their development as single molecule probes, particularly under the tightly focused excitation in single particle imaging. The surface plasmon resonance was tailored by application and variation of the thickness of a gold shell coating on the UCNP surface. This resulted in the single gold-shell coated nanoparticles showing enhanced absorption in the near infrared and enhanced total emission intensity. Quantitative modeling of both the excitation enhancement and the emission spectra shows where within the nanocrystal, the green/red emission enhancement occurs, and what portion of the enhancement is due to electromagnetic effects.

SEM of UCNPs (a) bare, (b) SiO2 coated, (c) SiO2/Au seeds, (d) SiO2/Au shell

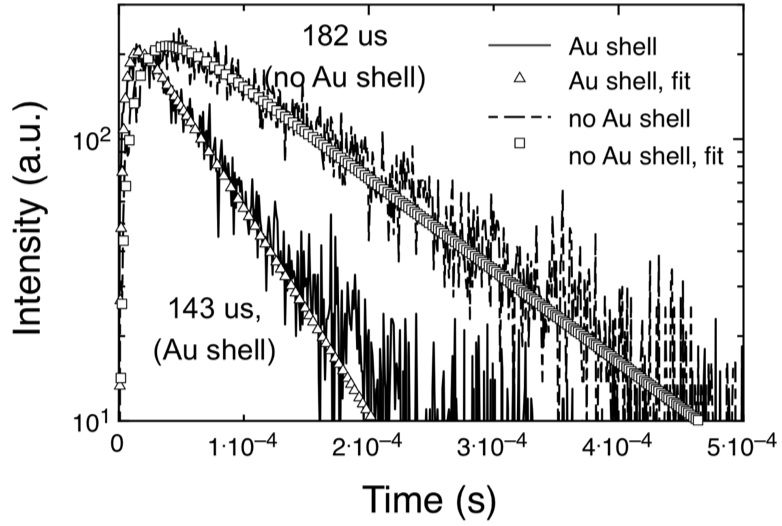
DNA Methylation Detection Using Resonance and Nano Bowtie Antenna
Enhanced Raman Spectroscopy
Methylation of cytosine to form 5-methylcytosine (5mC) is one of a number of epigenetic modifications that modulate DNA transcription activity without changing the DNA sequence. The global level of 5mC in human body has been suggested as not only a diagnostic biomarker indicating early tumors, but also useful risk assessment tool for clinical treatment of bacterial and viral infections. 5mC derivatives formed during the demethylation pathway are implicated in cancers and their progressions. I work in collaboration with the Hallen and Riehn groups in the determination of the methylation state of cytosine without any labels, additional reagents, nor sample amplification. We utilize resonance Raman measurements combined with a bow tie nano-antenna array, and excitation at wavelengths in the ultraviolet region to tune excitation wavelength to the absorption resonance of the targeted 5mC or non-methylated cytosine at the ring absorption resonance, in order to obtain high signal from DNA segments. From our measurements, we show distinct Raman spectral features attributed to methylation, at ~1000 cm -1 and from 1200 – 1700 cm -1.
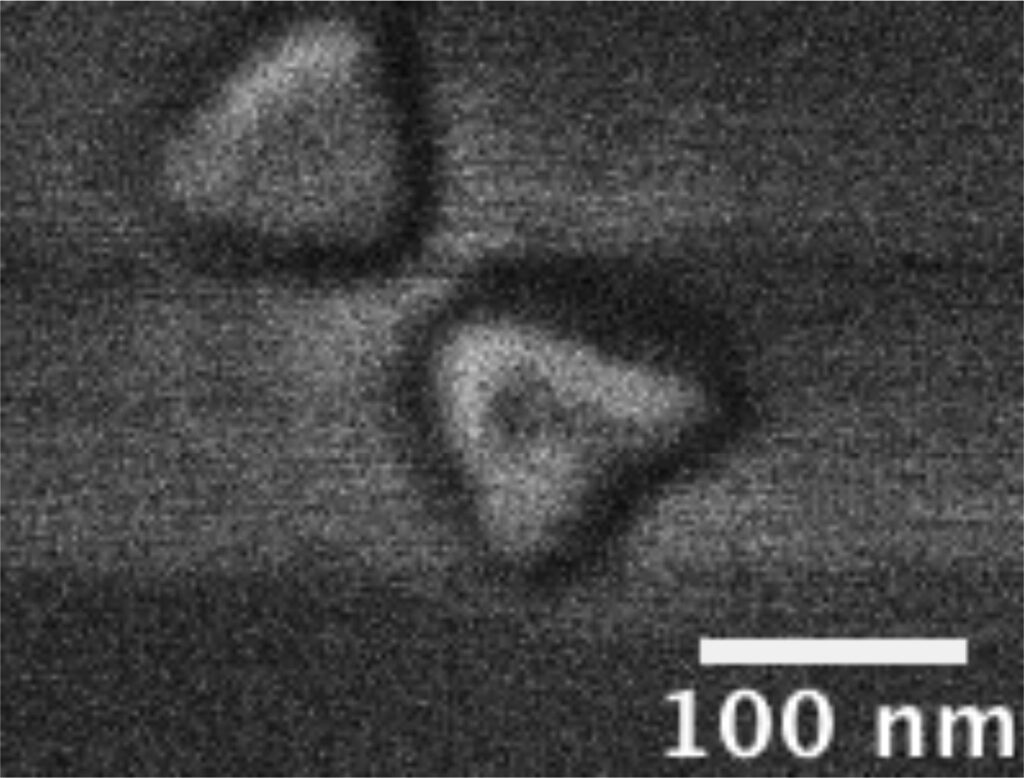

Both were acquired at 0.2 mW excitation at the sample, have been normalized, and the methylated DNA spectra reduced by a further factor of 10 to correct for resonance effects. The methylated spectrum has been
shifted upwards by 100 units for clarity
Funding
US ARMY ACC-APG-RTP W911NF (PI Lim)
National Science Foundation CBET (1067508, PI Lim)
National Institute of Health (1R21ES027641-01, PI, Lim)
National Institute of Health (1R21GM126887-01, co-PI Lim)
Collaborators
Dr. Hans Hallen, Physics, NCSU.
http://www.physics.ncsu.edu/people/faculty_hallen.php
Dr. Robert Riehn, Physics, NCSU.
http://www.physics.ncsu.edu/people/faculty_riehn.php
Dr. Hong Wang, Physics, NCSU.
http://www.physics.ncsu.edu/people/faculty_wang.php
Dr. Gang Han, Biochemistry, University of Massachusetts Chan Medical School
https://profiles.umassmed.edu/display/133316
Dr. Guanying Chen, Chemistry, University of Harbin
Leave a Reply